Luigi Ghezzi, Technical Marketing Engineer at Hamamatsu outlines how accessible sensing technology for Raman spectroscopy could help to revolutionise molecular analysis, from historical art authentication to pharmaceutical research
Spectroscopy, a field within the physical sciences, examines and employs the interactions between matter and electromagnetic radiation. The primary types of spectroscopy can be categorised based on whether we are measuring absorbed, reflected, emitted, transmitted, or scattered light of the sample, or if we are examining fluorescence.
What are the types of spectroscopy?
Spectroscopy is a multifaceted field, with the second step of characterisation further categorised according to wavelength range. This process includes various forms of spectroscopy, including X-ray, visible, infrared, and microwave. Hamamatsu offers high-performance components and modules for both the visible (300-700nm) and near-infrared (700-2500nm) range, which has become increasingly popular in chemical, material, environmental, and other types of analyses.
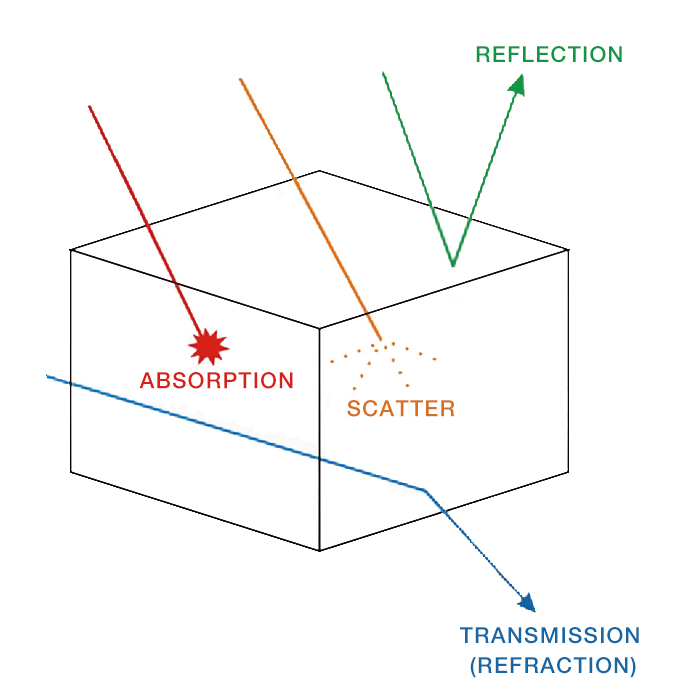
Figure 1: Types of spectroscopy
Visible spectroscopy absorption is a process by which atoms and molecules can absorb light in the visible range through electronic transitions. When an atom or molecule absorbs light of the right energy or wavelength, it will result in a decrease in the amount of transmitted light. In the condensed phase (liquids and solids), visible light absorption occurs mainly from complex conjugated double bonds and aromatic rings present in organic molecules. However, it is important to note that many aqueous transition metal ions can also form strongly light-absorbing species through complexions with associated water molecules in visible spectroscopy absorption. The diverse spectrum of hues that we observe in solids and liquids is a result of the absorption of light through electronic transitions. This process produces a broad array of colours that captivate our senses and leaves us in awe.
Within the near-infrared (NIR) spectrum, the energy present in the light is insufficient to activate the electronic transitions observed in the visible range. Consequently, the vividly coloured compounds that appear in the visible range exert less impact on the NIR. In the NIR range, absorption of light occurs through vibrational transitions. This type of absorption causes an increase in the vibrational mode between two atoms bonded together in a molecule when the light has the appropriate energy or wavelength. As opposed to the visible range, NIR absorption does not necessitate conjugated bonds or solvent complexes. Thus, it is more responsive to the molecule’s structure and its interactions with other molecules in the vicinity. This sensitivity to the molecular structure and environment accounts for NIR spectroscopy’s efficacy. One of the most important NIR Spectroscopy techniques is Raman Scattering.
Raman spectroscopy
Raman spectroscopy relies on the vibrational modes of the crystals or molecules being analysed. When light is directed at a material, some of it is scattered. The scattered light comprises both light with the same wavelength as the incident light (known as Rayleigh scattered light) and light with different wavelengths (known as Raman scattered light). Raman spectroscopy examines the properties of the Raman scattered light to identify the molecular or crystal structure of substances. This technique is suitable for analysing most substances, including inorganic compounds, organic matter, solids, liquids, gases, and more, without the need for special pre-treatment.
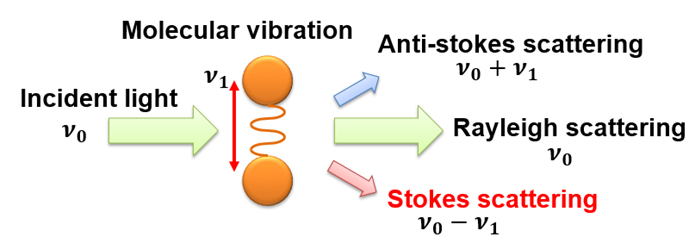
Figure 2: The principle of Raman spectroscopy
In Raman spectroscopy, the energy shift that occurs during Stokes scattering is referred to as the Raman shift, which is the parameter the technique aims to measure. However, Stokes scattering is significantly less likely to occur than Rayleigh scattering. Raman spectroscopy is a ‘fingerprint technique’, meaning that specific substances produce unique spectral signatures. The information obtained through Raman spectroscopy is diverse and rich.
Raman spectroscopy is particularly useful in the field of historical art authentication, as it can analyse the crystalline or molecular structure of paint to check its authenticity. Similarly, Raman spectroscopy is an excellent tool for analysing crystalline samples in the semiconductor industry, as well as in the chemical and pharmaceutical industries since it is a point-and-shoot technique that requires no sample pre-treatment. The information derived from Raman spectroscopy can be classified into four main categories.
Figure 3. Four Raman spectroscopy categories
The first is the energy shift, or Raman shift, which provides general information about the crystal or molecule in the sample. The second is the intensity, which indicates the concentration of each substance. Thirdly, it is analysing the shape of the peak and measuring the full width at half maximum to yield information about the crystalline structure. Lastly, potential peak deviations can provide insight into interactions with surrounding molecules, or they may be due to strain and distortion of the molecular structure.
We can easily see this for example in the spectrum of the different states of water in the figure below.
Figure 4: Spectrum of different states of water
We can see that the Raman signal of water vapour is a sharp peak, whereas liquid water and frozen ice have much broader peaks (due to their molecular interaction with the surrounding molecules).
One of the peculiar aspects of the Raman spectroscopy method is that this energy shift is independent of the excitation wavelength used. This means Raman spectroscopy can be performed across a broad energy range. Popular wavelengths are for example 532nm, 785nm, or 1,064nm (common semiconductor laser diode wavelengths).
Figure 5. Intensity measurement of methanol
Figure 6. Comparison of the Raman spectrum of pure Methanol and Ethanol
As shown in the figure above, the two spectra have similar peaks corresponding also to the same wavelength, but Ethanol has further peaks, which are distinct. This difference is because Ethanol is a larger molecule so there are more and different vibration modes that can be excited by Raman spectroscopy.
The examples shown previously demonstrate high-quality Raman spectra with excellent spectral resolution and signal-to-noise ratio. However, obtaining such high-quality spectra is not a trivial task due to the low probability of Stokes scattering compared to Rayleigh scattering, which is larger by factors of millions to billions.
A standard Raman spectroscopy setup consists of three key elements: a coherent light source (typically a laser), optical components such as mirrors, gratings, and filters, and a sensitive sensor for signal acquisition. The relevant spectral region for Raman spectroscopy is typically the visible and near-infrared part of the spectrum, and a detector with high sensitivity is required to detect the extremely faint Raman scattered light. Linear image sensors are commonly used to simultaneously detect multiple wavelengths that are dispersed.
Hamamatsu Photonics is a leading supplier of high-quality, high-performance, and affordable CMOS technology for industrial, analytical, and spectroscopy markets. With extensive experience in the field of photonics technology including optics, electronics, mechanics, and software, we offer a wide product portfolio including CCD (with TDI-CCD), NMOS, and CMOS.
Our new CMOS linear image sensor, S16596-4096-11. It builds upon the advantages of the previous model, with a longer pixel size (7x200 µm), 4096 pixels, a maximum data rate of 10 MHz, low readout noise (typ. 0.4 mV rms), high dynamic range (5000 times). It includes key functions such as simultaneous charge integration for all pixels, variable integration time, an electronic shutter, and a built-in timing generator. The S16596-4096-11 sensor also boasts higher sensitivity, making it an excellent choice for Raman spectroscopy applications.
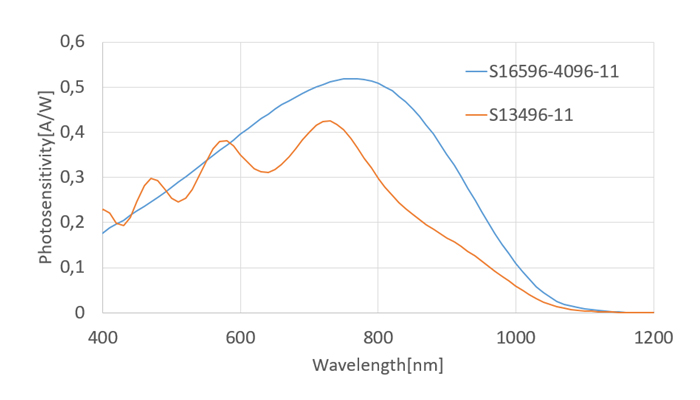
Figure 7. Comparison with the previous model
In addition to the sensor, we can provide a driver circuit that offers a 16-bit digital output and is compatible with USB 2.0 interfaces. The power supply is DC+5V, which can be provided via the USB bus power. The readout frequency of the driver circuit is 10MHz, and the size of the Sensor Board is 20 x 41.6 mm, while the Interface Board measures 45 x 50 mm. In addition, the external synchronisation operation is available with this driver circuit.
If you want to test this CMOS for your application, an evaluation board comprising sensor and readout boards, and lens mount (F-mount) is available.
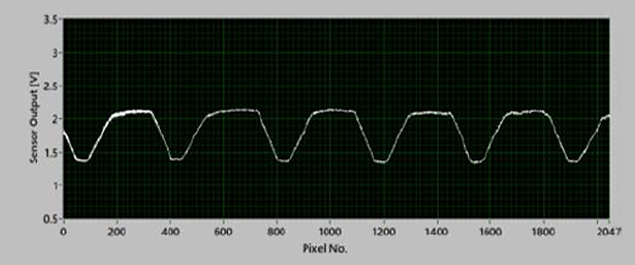
Figure 8. Typical output of the readout board The vertical axis indicates the sensor output. The horizontal axis indicates the number of pixels
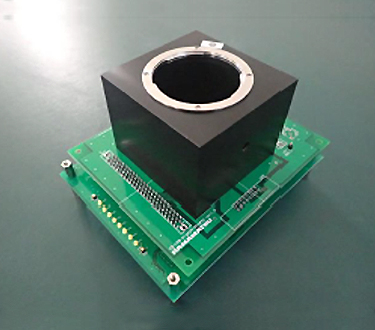
CMOS linear image sensor, S16596-4096-11