Gaetano Panagia, Technical Marketing Engineer at Hamamatsu Photonics Europe, explains how to detect certain gases by identifying their specific absorption wavelengths in the mid-infrared spectrum. This article covers the practical application of infrared spectroscopy using non-dispersive infrared (NDIR) gas measurement. It outlines the typical design of NDIR gas detectors, introducing several measures that can be adopted to mitigate the challenges faced by these detectors. The different configurations of NDIR gas detectors, such as single-beam and dual-beam are explained, together with the advantages of using LED emitters. The article also introduces an experimental setup that successfully measured CO2 concentration from 0 to 5000 ppm using a Hamamatsu Photonics’ Mid-IR LED and an InAsSb detector.
Infrared spectroscopy is a method that relies on the vibrations of a molecule's atoms. It involves passing infrared radiation through a sample and analysing how much of the radiation is absorbed at a specific wavelength. This creates an infrared spectrum which shows different characteristics for each specific sample, the sample's infrared “fingerprint”.
One practical application of infrared spectroscopy is non-dispersive infrared (NDIR) gas measurement, which originated in the late 1930s in the United States. NDIR gas measurement focuses on detecting certain gases by identifying their specific absorption wavelengths in the infrared spectrum. This technology is particularly useful for identifying air pollutants emitted from various sources, such as carbon monoxide (CO), carbon dioxide (CO2), sulfur dioxide (SO2), nitrogen oxides (NOx), nitrous oxide (N2O), ammonia (NH3), hydrogen chloride (HCl), hydrogen fluoride (HF), methane (CH4), and more.
NDIR sensors provide exceptional long-term stability and high gas specificity. For example, when it comes to CO2 sensing, traditional non-optical methods face considerable challenges in terms of detection speed, accuracy and frequent maintenance requirements, making NDIR a particularly well-suited technique.
The significant absorption strength of CO2 in the mid-IR region opens new possibilities and pathways for accurate CO2 detection using NDIR technology.
An NDIR spectrometer generally consists of a gas chamber, a light emitter, and a detector. Nevertheless, open path systems, which do not include a gas chamber, are widely used in fenceline monitoring and leaks detection, where gas emissions need to be measured over long distances.
While their advantages remain undeniable, these detectors are by no means immune to encountering substantial challenges. Among the most widespread obstacles is the interference of signals due to water absorption in environments with elevated humidity levels. To combat this issue, a common approach involves employing light sources that emit in the mid-infrared range rather than the near-infrared range. This choice is rooted in the fact that longer wavelengths experience reduced impact from water absorption. Alongside this, NDIR gas detectors grapple with additional hurdles including low signal-to-noise ratios, which can obscure precise gas measurements and elevate the Limit of Detection (LOD). Signal drifts also pose a concern, arising from fluctuations in temperature, power supply, or the aging of emitters. This article elucidates the fundamental principles of NDIR spectroscopy, outlines the typical design of NDIR gas detectors, and introduces several precautionary measures that can be adopted to mitigate the aforementioned challenges.
General principle
During NDIR measurement (Fig.1), the IR light in the gas chamber is absorbed by the target gas, and this absorption is quantitatively described by the Beer-Lambert law:
I= I0 e-εcL (1)
in which I and I0 are the light intensity signals measured in presence and absence of the target gas, respectively,
𝜖 is the molar attenuation coefficient of the target gas, c is the target gas concentration, and L is the interaction length. From Equation (1), one can calculate the transmission T as:
T= I/I0 = e-εcL (2)
The relationship between the transmission from the source to the detector (T) and the target gas concentration in the gas chamber can be observed for a specific sensor configuration (fixed L). Hence, it becomes feasible to determine the gas concentration by measuring the changes in transmission from the source to the detector.
NDIR gas detector configurations
Single beam configuration
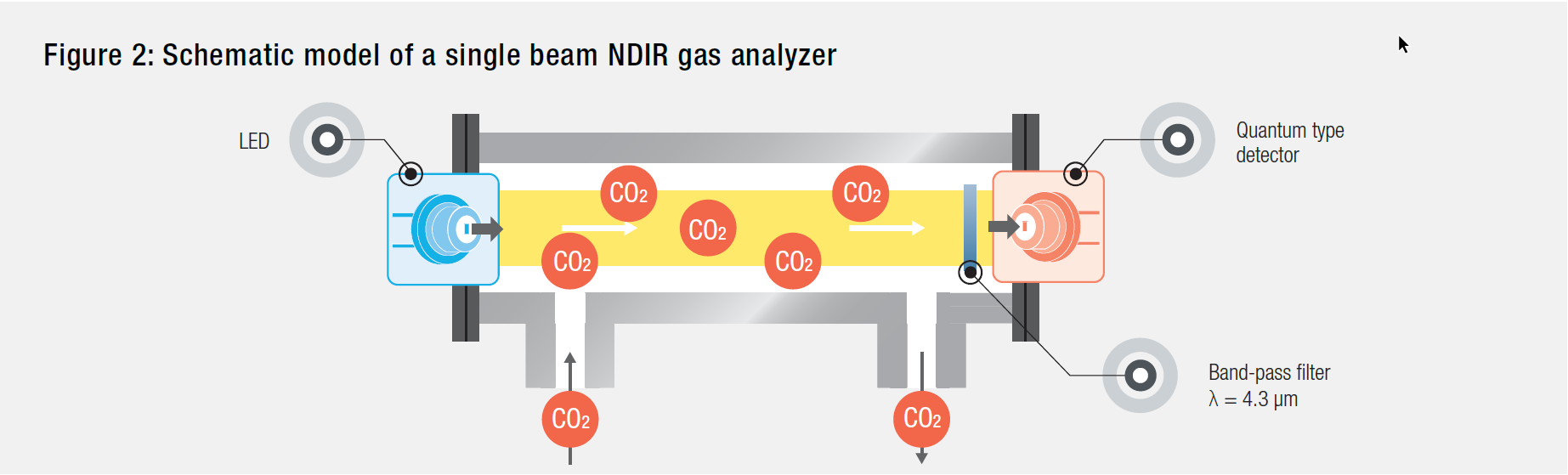
The simplest NDIR gas detector uses an IR lamp or a LED source, gas chamber, and a single optical detector. The latter can be a thermal light detector, such as a thermopile, or a quantum type, such as an InAsSb detector. The choice between thermal or quantum type detectors depends on the application speed and sensitivity requirements, as summarized below in Table 1.
An NDIR CO2 measuring setup was built using Hamamatsu’s Mid-IR LED evaluation kit M16615, InAsSb evaluation kit M16953, a gas pipe with a length of 200mm and internal diameter of 5mm. The emission wavelength of the Mid-IR LED and the centre wavelength of the bandpass filter, installed over the InAsSb detector, are compatible with the absorption wavelength of CO2 at 4.26 µm.
The results obtained with this setup are shown in Fig.4 and Fig.5 below:
This experimental setup successfully measured CO2 concentration from 0 to 5000 ppm and a 36% signal reduction was observed, caused by the absorption of CO2 in the gas chamber. The obtained values are compatible with the typical CO2 concentration in the atmosphere, in a well-ventilated room and in a poorly ventilated one (Table 2).
Dual beam NDIR sensors
If the application requires higher accuracy, the NDIR gas sensor design can be improved by adopting two optical detectors. These detectors serve as the sensor's two channels: the sensing channel and the reference channel. Optical filters in front of the detectors enable wavelength selection for each channel. The sensing channel detection wavelength overlaps with the absorption band of the gas of interest, while the reference channel wavelength is not absorbed by the gas. For CO2 sensing, wavelengths around 4.26 μm and 3.9 μm are commonly used for the sensing and reference channels, respectively.
In this configuration, the signal produced by the sensing channel can be normalised using the signal from the reference channel.
The presence of the reference channel plays a crucial role in compensating for light intensity variations caused by factors including source power fluctuations, wavelength shifts due to temperature changes, and detector common mode noise. This compensation allows for auto-calibration of the sensor.
Dual channel detectors - P16849- 012CF
Hamamatsu Photonics provides dual channel detectors such as the P16849-012CF, which is designed for CO2 sensing. These special InAsSb detectors are optimized for dual beam NDIR sensors: they are extremely fast, highly sensitive and are equipped with customisable bandpass filters.
An alternative approach for the compensation of light intensity variations is to introduce an absorption bias between the signal and reference channel outputs. This bias is achieved with a variation in the design of the gas chamber, by employing a longer path length for the signal channel compared to the reference channel. Both the signal and reference detectors are equipped with identical spectral filters that overlap with the gas's absorption band to be measured[5]. In fact, considering the Lambert-Beer relation (2), when the target gas is not present in the chamber, the target gas concentration c is approximately zero. In this situation I = I0 in both the sensing and reference channel. When the target gas is introduced into the NDIR sensor, the values of 𝜖 and c will be the same for the two arms of the device, while the path length of the sensing channel will be much longer than the one in the reference channel, thus generating a difference in the two signals.
An additional method to improve signal-to-noise ratio in a NDIR gas detector is using lock-in amplification. Columbine Robinson has shown that this technique can improve SNR up to 5.3 fold in the technical note “Noise mitigation strategies for infrared detection”.
Mid-IR LEDs for NDIR gas detectors
LEDs (Light-Emitting Diodes) are the preferred light source in the design of NDIR (Non-Dispersive Infrared) gas detectors for several reasons:
- Wavelength Selection: LEDs can be designed to emit light at specific wavelengths that correspond to the absorption bands of the target gas molecules. This allows for selective and accurate detection of specific gases.
- Compact Size: LEDs are small and compact, making them suitable for integration into portable or handheld gas detectors. Their small size also enables the construction of multi-channel gas sensing systems with multiple LED sources for detecting different gases simultaneously.
- Low Power Consumption: LEDs operate at low power levels, which is advantageous for battery-powered or energy-efficient gas sensing applications. They require less power compared to other light sources like incandescent lamps or lasers, resulting in prolonged battery life and reduced energy consumption.
- Rapid Response Time: LEDs have fast response times, enabling quick measurements and real-time monitoring of gas concentrations. This is particularly important in applications where rapid detection and response to changing gas levels are required, such as industrial safety systems or environmental monitoring. In addition, high frequency emission enables lock-in amplification techniques used to improve the measurement SNR.
- Long Lifespan: LEDs have a long operational lifespan compared to traditional light sources, reducing maintenance requirements and overall cost of ownership.
- Cost-Effectiveness: LEDs are relatively inexpensive compared to other light sources like lasers or thermal emitters. This makes them a cost-effective choice for mass production of NDIR gas detectors.
In addition, mid-infrared wavelengths are the most common choice for NDIR gas detectors for three main reasons:
- The absorption peaks of many popular target gasses are higher in the mid-infrared range compared to visible and near-infrared, enabling a higher sensitivity of the detector.
- These absorption peaks are better spaced apart in the mid-IR spectrum, leading to lower cross- sensitivity and better gas selectivity.
- Water shows a lower absorbance for mid-IR wavelengths, thus interfering less with the measurement, especially in open path detection.
Hamamatsu Photonics offers a wide range of LEDs emitting in the mid-infrared spectrum. These are available in several different wavelengths and packages for every NDIR application need.
Specifically, Hamamatsu Photonics has recently released some SMD LEDs emitting at 3.3, 3.9 and 4.3 µm. These are designed to minimize footprint and are easy to integrate into portable NDIR analysers.
SMD LED - L15895-0430CN
SMD LED - L15895-0430CN
LED with integrated reflector - L15895-0430ML
Conclusion
NDIR technology provides a robust method to accurately detect and quantify gases like CO2 by measuring transmission changes from the source to the detector. We proposed various NDIR sensor designs, including single-beam and dual-beam configurations, with the latter offering higher accuracy through auto-calibration and compensation for light intensity variations. Additionally, the article underscores the benefits of mid-infrared (MIR) LEDs as preferred light sources for NDIR gas detectors, thanks to precise wavelength selection, compact size, low power consumption, and fast response times. Hamamatsu Photonics' range of MIR LEDs and detectors contribute significantly to NDIR gas measurement advancements, offering reliable and economical solutions. These technologies drive sustainable growth and environmental stewardship, paving the way for a brighter, cleaner, and safer future in various applications, such as industrial safety, environmental monitoring, and breath analysis.
Bibliography
- [1] T. Dinh, I. Choi, Y. Son, J. Kim, "A review on non-dispersive infrared gas sensors: Improvement of sensor detection limit and interference correction," Sensors and Actuators B: Chemical, vol. 231, pp. 529-538, 2016. ISSN 0925-4005. [Online] Available: https://doi.org/10.1016/j.snb.2016.03.040.
- E. Naranjo and S. Baliga, "Early detection of combustible gas leaks using open path infrared (IR) gas detectors," Proceedings of SPIE - The International Society for Optical Engineering, vol. 8366, p. 27, 2012. DOI: 10.1117/12.919201.
- Kim Soocheol, Park Soyoung, and Lee Kangbok, "Method for Aerosol Particle and Gas Analyses based on Dual- channel Mid-infrared Sensor," Int J Fire Sci Eng, vol. 36, no. 1, pp. 1-6, 2022. DOI: 10.7731/KIFSE.3d1404d5.
- X. Jia, J. Roels, R. Baets, and G. Roelkens, “A Miniaturised, Fully Integrated NDIR CO2 Sensor On-Chip,” Sensors, vol. 21, no. 16, p. 5347, Aug. 2021, doi: 10.3390/s21165347.
- J.Y. Wong and M. Schell, "Zero drift NDIR gas sensors," Sensor Review, vol. 31, no. 1, pp. 70-77, 2011 [Online] Available: https://doi.org/10.1108/02602281111099116.
- "Mid-Infrared Light Emitting Diode (LED) Light Source: Applications in Mid-Infrared Spectroscopy," U.S. Patent US 2011/0042570 A1, February 24, 2011.
- Columbine Robinson, "TECHNICAL NOTE: Noise Mitigation Strategies for Infrared Detection", Hamamatsu Corporation, June 2023. [Online] Available: https://www.hamamatsu.com/content/dam/hamamatsu-photonics/ sites/documents/11_HC/21_HC_documents/Tech%20Note-LIA-final.pdf